Comparison of Different Materials Used in The Preparation Of Household Bioplastics and Evaluation of a Mixture of Animal Gelatin and Cornstarch
2025-01-23 | Volume 3 Issue 1 - Volume 3 | Research Articles | Kamar Shayah | Joseph Shenekji | Durra Balt | Siran Arabanian | Joudy Awyga | Sawsan SalloumAbstract
The harmful environmental impacts of plastic materials used in packaging have necessitated the adoption of environmentally friendly bioplastics as an alternative to petroleum-derived plastics due to their unique properties. While most bioplastics are starch-based, they are not without shortcomings, thus requiring improvements in their mechanical and chemical properties. In this study, experiments were conducted with various materials to produce bioplastics to evaluate the effect of these materials on the final product’s consistency. In the final experiment, the combination of corn starch and animal gelatin was tested for its impact on the mechanical and chemical properties of the final product. The impact of incorporating animal gelatin into corn starch on the chemical and mechanical properties of the end product was examined. The resulting product exhibited improved texture and tensile strength compared to membranes made from starch alone. Microscopic analysis revealed that the final product had a denser texture and more granular appearance than the starch-based products. By evaluating the product characteristics, it is evident that this material can be utilized for household food packaging due to its favorable tensile and mechanical properties at a cost-effective price. Several bioplastic mixtures were compared in this study. The results indicate that the optimal bioplastic composition consists of a blend of corn starch 2.6% and animal-derived gelatin 5.2%. This hybrid material demonstrated superior mechanical properties, biodegradability, and overall functionality compared to the pure constituent components. The synergistic effects observed in the corn starch-gelatin bioplastic suggest that this formulation represents a promising sustainable alternative to traditional petroleum-based plastics. It can be used and manufactured domestically and industrially, and it showed long durability and decent expiration before degradation. Further research is recommended to explore the scalability and potential industrial applications of this innovative bioplastic.
Keywords : Bioplastics, Corn Starch, Animal Gelatin, Glycerol.
INTRODUCTION
Bioplastics are biodegradable materials derived from renewable sources and offer a sustainable solution to the problem of plastic waste. Unlike traditional plastics, they are not derived from non-renewable fossil resources such as oil and gas[1]. Bioplastics can be made from a variety of natural materials such as agricultural waste, cellulose, potatoes, corn, and even wood powder. These materials are converted into bioplastics through various preparation processes that exploit existing plastics manufacturing infrastructure to produce bioplastics that are chemically similar to conventional plastics, such as bio polypropylene [2]. A common type of bioplastic is polyhydroxyalkanoate (PHA), a polyester produced by fermenting raw plant materials with bacterial strains such as Pseudomonas. It is then cast into molds for use in various industrial applications, including automotive parts. Bioplastics have many advantages such as carbon footprint, which is defined as the total amount of greenhouse gases (GHGs), specifically carbon dioxide (CO2) and methane (CH4), emitted directly or indirectly by an individual, organization, event, or product throughout its life cycle. This measurement is typically expressed in equivalent tons of CO2 reduction, energy saving in production, avoiding harmful additives such as phthalate or bisphenol A; being 100% biodegradable, bioplastics have applications in sectors such as medicine, food packaging, toys, fashion, and decomposable bags [3][4]. Bioplastics are characterized by their environmentally friendly nature and their ability to reduce plastic pollution. While they offer a promising alternative to traditional plastics, there are challenges related to production and land use costs, energy consumption, water use, recyclability, etc. Ongoing research aims to develop more environmentally friendly types of bioplastics and improve production processes for a more sustainable future, especially at the domestic level and not just at the industrial level [5].
Comparison of bioplastics with traditional plastics in terms of cost and durability
Bioplastics have a lower carbon footprint than synthetic plastics, help in the reduction of CO2 emissions, save fossil fuels, and eliminate non-biodegradable plastic waste. [6] [7] Conversely, traditional petroleum-based plastics from non-renewable resources have a higher environmental impact and do not biodegrade. This petroleum based plastics contribute significantly to pollution that persists in the environment for hundreds of years, causing pollution and damage to ecosystems. While traditional plastics are durable, low-cost, and waterproof, they face challenges due to their slow degradation and negative environmental impacts, especially on marine life where most plastic waste resides [6]. When comparing cost and durability, bioplastics may face production cost challenges compared to conventional plastics, due to factors such as the industrial refining of polymers from agricultural waste [8]. In terms of cost and mechanical durability, the production of bioplastics is more expensive than the production of conventional plastics, which is one of the challenges facing the production of bioplastics at the local and even global level. For example, their production from agricultural waste requires additional costs due to the necessary and multiple stages of refining and isolating the industrial polymers to be used in production. In addition, not all bioplastics have the same durability, thermal stability, and waterproofing properties as conventional plastics. However, many current researches are focused on developing bioplastics that can compete with traditional petroleum-based plastics.
History of the bioplastics industry
The bioplastics industry dates back to the early 20th century when companies began producing bioplastics as an alternative to traditional petrochemical-based plastics. [5][9].
The first attempt to produce bioplastics was in 1862 when Alexander Parkes produced the first man-made plastic called Parkesine. It was a biological material derived from cellulose that, once prepared and molded, maintained its shape as it cooled [10] [11] [9].
Production Methods
Bioplastics are made using various processes, including the conversion of natural materials into polymers suitable for commercial use.
These processes include microbial interactions and nanotechnology synthesis methods, such as crystal growth and polymer extraction from microbes. Using raw materials such as cornstarch, sugar cane, vegetable oils, and wood powder are common in bioplastics production [12].
Current challenges and prospects
The environmental benefits of bioplastics offer advantages such as reducing the carbon footprint, saving energy in production, and avoiding harmful additives found in conventional plastics [13]. Bioplastics represent a promising alternative to traditional plastics due to their lower environmental impact; however, their production costs remain higher, which limits their market adoption. Recent research is focused on improving production efficiency and reducing costs through innovative technologies and sustainable practices. These efforts include utilizing microbial processes, enhancing fermentation techniques, and leveraging agricultural waste as raw materials. By addressing these economic challenges, bioplastics can become a more viable option, contributing to environmental protection and reducing dependence on fossil fuels. Ultimately, the advancement of bioplastic production will play a crucial role in promoting sustainability and mitigating the adverse effects of plastic pollution[14][15] . Many agree that improving the material properties of bioplastics is important for wider adoption and market competitiveness [16].
Benefits of using bioplastics
Bioplastics offer a multitude of advantages over traditional plastics, positioning them as a more sustainable and eco-friendly alternative to mitigate the carbon footprint associated with plastic manufacturing. Moreover, they help to reduce the consumption of fossil fuels [17]. Bioplastics exhibit accelerated decomposition rates, decomposing within a span of a few months in stark contrast to the centuries required for conventional plastics to degrade[18]. This rapid degradation contributes significantly to mitigating environmental pollution and minimizing the presence of microplastics in ecosystems. Notably, bioplastics are derived from renewable resources, supporting sustainable practices by utilizing annually replenishable materials[11]. Bioplastics are considered toxin-free, because they are derived from natural materials and do not contain harmful or toxic chemicals commonly found in conventional plastics, and they degrade without releasing harmful substances [19]. Bioplastics can be fully recyclable and biodegradable, providing a closed system for maximum sustainability impact, they provide improved waste management solutions by reducing the number of plastics sent to landfills and encouraging recycling practices [20] [21].
MATERIALS AND METHODS
Main chemicals used in bioplastic manufacturing
Animal Gelatin, Acetic Acid, Glycerin, Corn Starch
Animal Gelatin: Food-grade animal gelatin, derived from collagen, [22] was used in this study due to its excellent techno-functional properties, including water binding and emulsification. It can be produced domestically by soaking animal bones in hydrochloric acid to remove minerals, followed by extensive washing to eliminate impurities[23] . The cleaned bones are heated in distilled water at 33°C for several hours, then extracted and placed in water at 39°C for further extraction[24]. The resulting liquid undergoes chemical treatment to produce pure gelatin, which is then concentrated, cooled, cut, and dried to achieve optimal quality and gel strength. [25] [26]
Acetic Acid: Acetic acid is utilized in the production of polyethylene terephthalate (PET) and polyvinyl acetate (PVA). It serves as a solvent in oxidation reactions and enhances the properties of plastics, such as elasticity and transparency. [27]
Glycerin: Glycerin is a non-toxic, water-soluble polyol compound that provides flexibility and mechanical strength in bioplastics while enhancing texture and material stability[28].
Corn Starch: Corn starch, composed of amylose and amylopectin, serves as a carbohydrate reserve for plants[30] [29]. Its extraction involves cleaning the grains (Zea mays saccharata),[31] soaking them in a dilute sulfur dioxide solution at 47°C with a pH of 3.5 for 48 hours, followed by crushing, sedimentation, washing, and drying to obtain powdered starch. [32]
protocol for making bioplastics from starch and animal gelatin
First, 1.5 g of cornstarch was weighed using an accurate balance (or equivalent to 3% of the weight/volume of the final mixture), then 3 g of commercially available animal gelatin powder used in the manufacture of sweets is weighed using an accurate balance (or equivalent to 6% of the weight/volume of the mixture). These chemicals are dissolved in 50 ml of tap water (or the volume that achieves a ratio of 3% cornstarch and 6% animal gelatin) at room temperature and, while stirring by a small magnetic stirrer to ensure complete dissolution of the gelatin and homogeneity of the solution, 1.5 ml of pure glycerin is added, then 1.5 ml of commercial acetic acid is added using a graded cylinder. The mixture was gently stirred until the ingredients were homogeneous, then placed in a microwave oven [33], covered with a thin cloth on top, and turned on for 2 minutes, checking the thickness of the mixture every 30 seconds. The microwave oven is turned off until the boiling foam disappears, then heating is continued until the end of the two minutes. After removing the mixture from the microwave oven, the mixture is cooled with running tap water by letting water stream on the outside of the bowl until the temperature of the bowl reaches 60° C. The resulting mixture is poured directly into a Petri dish or other suitable surface and spread out until its dimensions are homogeneous and its thickness is uniform over the entire surface, preferably 4 mm of height in a regular Petri dish. Finally, the mixture is left at room temperature until it hardens, which can take 16-24 hours or longer depending on the thickness and exposure to higher temperatures. In this study, seven mixtures of bioplastics with different materials were prepared and compared, including: potato starch, corn starch, wheat starch, animal gelatin, plant-based gelatin (agar-agar), animal gelatin with cornstarch, and animal gelatin with plant-based gelatin (agar-agar), if we counted also the solvents and enhancements like adding wax to make the bioplastic waterproof and antibiotic and antifungals to make it resistant to bacteria and mold, there would be 22 different compositions tested in this study, the table of these tests is provided in table s1 in supplementary materials.
RESULTS
The evaluation of the bioplastic mixtures revealed that the optimal formulation was a combination of corn starch and animal gelatin. Upon solidification, this mixture produced a cohesive bioplastic with a flexible texture and sufficient strength, making it suitable for a range of packaging applications. Notably, this bioplastic is capable of decomposing in soil within a period of 3 to 4 months or when immersed in water for approximately 3 weeks. Furthermore, it can retain its functional properties for up to 6 months when stored at room temperature and shielded from moisture.
Moisture content (MC)
The moisture content of the plastic was calculated by comparing the initial weight (W1=2 mg) of the bioplastic film (2 cm × 2 cm) and the final weight (W2=1.5 mg), which was determined after a 10 min oven-dry period at 120° c, as shown in equation 1. The result showed that the moisture content was 25%, and the shape of the results is shown in Fig. 1.
Fig1. A comparative image of a bioplastic sample before and after heating in an oven with visually observed changes.
The density of the bioplastic
The density of the bioplastic film (2 cm × 2 cm) was determined by measuring the mass (M) and area (A) of the known bioplastic film thickness (d) using equation 2, and the density was 0.16 g/cm-3 for the cornstarch and animal gelatin mixture bioplastic.
Density = M/A×d [35]
Density of corn starch and animal gelatin mixture = 12/50.24×1.5= 0.16 g cm−3
Water solubility
The sample of cornstarch and animal gelatin mixture (2 cm x 2 cm), was submerged in 30 ml of water for 48 hours at 27 ° c, and no complete dissolution of the sample was observed, indicating that the bioplastic film did not fully dissolve in the water. It has lost approximately 50% of its total weight. However, the flexibility and tensile strength of the sample were observed to have changed. The bioplastic film, which was initially flexible, became coarse and lost its ability to withstand tensile stress, resembling the properties of non-tensile nylon, in a simple finger touch evaluation. [36] The samples made from cornstarch partially dissolved in water, whereas the sample made from animal gelatin transformed into a gel-like consistency. Meanwhile, the sample composed of both starch and animal gelatin altered its texture to resemble that of nylon. [37] Adding 1g of melted wax to overcome water solubility for longer periods resulted in crumbly textures and heterogeneity due to the incompatibility of starch with wax. However, applying dry wax to the surface by rubbing on the solid bioplastic film showed better results, but the water tolerance was acceptable in terms of time (3 months) without the wax.
Biodegradability
A 4 cm x 2 cm sample of the bioplastic was placed in red soil and exposed to the outdoor environment. The soil was watered once a week for the first three weeks to mimic agricultural water exposure. After this time, it was observed that the sample had lost a small amount of its flexibility. The average temperature during this initial three-week period was 22o c in the morning and 10o c at night. In the sixth week, the soil was watered every 4 days, and the temperature increased to 24o c in the morning and 13o c at night. After 4 weeks of this experiment, it was found that the bioplastic had become brittle and fragile, with no flexibility remaining. Additionally, a noticeable reduction in the thickness of the sample was observed as shown in fig2. [38] To prevent mold, 0.5g of antifungal clotrimazole was added at a concentration of 100 ml of the mixture at 30o c. This showed fragility in texture, non-homogeneity, difficulty in drying the sample, and incoherence, as shown in Fig Image 7.
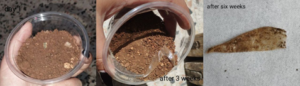
Comparison of different materials used to make bioplastics
After trying different mixtures and methods of making bioplastics, the following results were observed; The Potato starch mixtures did not give good results as the samples were fragile, brittle, and gave low durability. Corn starch mixtures gave good results in terms of durability and flexibility but didn’t withstand tension and pressure. Wheat starch also showed good results. The resulting plastics are flexible and can withstand light and heavy tension and pressure. Animal gelatin provides a hard plastic texture suitable for packaging but does not withstand moderate tension. Plant gelatin (agar-agar) yielded unsatisfactory results, the resulting plastics are fragile and lack cohesion and do not withstand light pressure. Animal gelatin mixed with cornstarch achieved the best results in terms of durability, consistency of texture and ability to withstand medium to high pressure. The addition of the antifungal clotrimazole (each 1g of product powder contains 10 mg of clotrimazole (1-Orto chloro-benzyl imidazole)) resulted in inconsistent texture and poor outcomes. Adding a layer of antibiotic (ampicillin 500 mg dissolved in 5 ml) by using a cotton spreader created a stiff texture in the bioplastic. A view of most of these results is shown in fig3 as follows: 1) animal gelatin, 2) corn starch, 3) wheat starch, 4) animal gelatin & potato starch, 5) potato starch, 6) animal gelatin & potato starch & wax, 7) corn starch & antifungal , 8) wheat starch & antifungal & wax, 9) corn starch & antibiotic, 10) plant gelatin (agar-agar) & antibiotic, 11) plant gelatin (agar-agar), 12) plant gelatin (agar-agar) & animal gelatin, 13) plant gelatin (agar-agar) & corn starch, 14) corn starch & wax, 15) plant gelatin (agar-agar) & wheat starch.
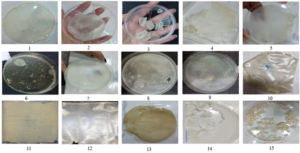
Transparency
The bioplastic made from animal gelatin and also the mixture of animal gelatin with corn starch showed the best results in transparency, and the transparency was different according to the thickness of the liquid mixture before solidifying. Transparency test is shown in Fig4.
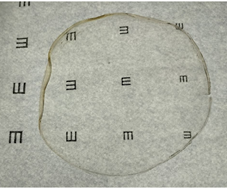
Microscopic structure
Examining the bioplastic made from the mixture of animal gelatin and corn starch under the microscope showed consistent appearance of several groups of units with few borders that were more permeable to light as shown in fig5.
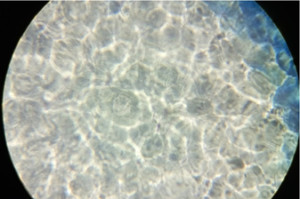
All the properties and results of the different mixtures are summarized in Table 1, which gives an estimated evaluation of each mixture used in the study in general, without additives such as wax and antibiotics and antifungals.
This study aimed to compare the effectiveness of different types of bioplastics made domestically, the properties of bioplastics varied greatly between materials, this can be beneficial in some aspects to use a type that gives a certain property in a desired application. Although wheat starch is cracked, it still has a rubbery and spongy texture that can be tested for shock absorbance and transfer fragile materials. Biofilms that were made from animal gelatin can be tested to be used in making bags.
Moisture Content: This mixture demonstrated a large decrease in moisture content when placed in the oven, losing about 75% of its moisture content. This could render this plastic unsuitable for most applications as the resulting water absorption would alter the properties of the plastic and reduce its tensile strength. Therefore, it is recommended that future studies be conducted to increase the moisture content. [39]
The density of the bioplastic: Plastic density influences the arrangement of molecular chains and intermolecular forces. Higher density indicates more tightly packed molecular chains and stronger intermolecular forces, resulting in greater strength and hardness. In contrast, lower density plastics have looser molecular arrangements and weaker intermolecular forces, enhancing flexibility, impact resistance, and transparency. This mixture demonstrated low density, which makes it suitable for use in packaging and plastic bags.
Biodegradability: The loss of moisture mentioned in the results show that this bioplastic can degrade over time in the absence of humidity, but also the water solubility property can make this plastic also degradable in aquatic environments, also soil exposure in the presence of water indicated by the biodegradability results showed that this bioplastic can be released into the environment and degraded in a matter of months.
Adding antifungal and antibiotic: Adding antifungal and antibiotic to the bioplastic showed that mixing the additive with the bioplastic created a fragile texture, while rubbing the additives on the dry bioplastic gave better results. The transparency of the bioplastic can be obtained by altering the thickness of the film and essentially having animal gelatin in the mixture, and the more you have a higher percentage of animal gelatin the more transparent your biofilm can be. Mixing animal gelatin with corn starch provided several benefits of the separate biomaterials, where cornstarch gave the rubbery structure and animal gelatin gave rigidity and transparency, and this mixture was selected as the best based on its stability and texture, yet more rigorous tests are recommended to further evaluate this bioplastic. The Protocol provided in this study is simple and can be used domestically and upscaled commercially to make bioplastic more available and integrated into daily culture.
CONCLUSIONS AND RECOMMENDATIONS
In this study, several biomaterials were investigated to make bioplastic domestically, to achieve economic efficiency and sustainability. Among the different combinations tested, the most promising results were obtained from the combination of animal gelatin and cornstarch. This particular formulation exhibited properties comparable to petroleum-based plastics, making it a suitable option for several domestic applications. Other mixtures were neglected and not further tested because of their breakage and dissociation. The protocol described in this research can be tested and improved to create even novel mixtures of bioplastics derived from animal gelatin sources such as bones from butchers or slaughterhouses and corn starch from crop residues, we were able to make a few yet to have an abundant quantity for tests we had to buy commercial gelatin. This study is considered initial research, and further rigorous tests of this gelatin-corn starch bioplastic are recommended, such as an FTIR test, mechanical property curves, in-vitro fungal tests to obtain more concrete results. possible implementation for use in greenhouses instead of oil-based plastic for it to be dissolved in the soil and become a fertilizer; with the benefit of protection from bioplastic contamination, or in packaging materials intended for food, where transparency and tamper-resistance are essential, but applications of packaging jewelry or small electronics like headphones or book leathering is very much possible with this mixture, all these applications needs further exploration. Bioplastic manufacturing is very feasible on a domestic approach, and it can reduce the use of petroleum-based plastic if adopted by families and societies whenever it is applicable, therefore we recommend its use and further studies on its application in several fields in packaging and preservation. We also recommend studying other forms of hard bioplastic to manufacture rigid bioplastic alternatives to regular plastic, such as biodegradable forks, knives, plates, etc.
References :
The authors declare there is no competing interest. Author contributions: All authors contributed equally. Data and Materials Availability: All data and materials used in the analysis are available in the article and supplementary materials, and further information could be provided upon contact with the corresponding author. Supplementary Materials: Supplementary table s1 contains different mixtures and additives to make bioplastic used in this research.
Competing Interests :
(ISSN - Online)
2959-8591